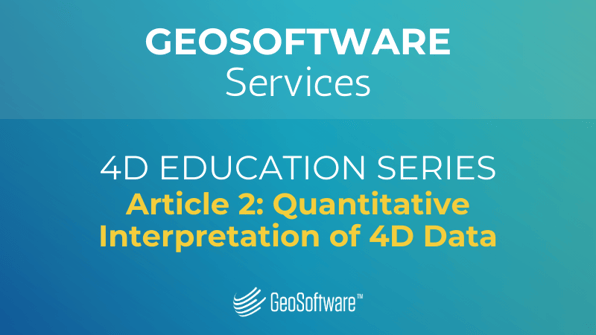
This is the second article of a three-part series dedicated to a review of seismic monitoring 4D. The series describes how valuable 4D techniques can be achieved and realized through the application of GeoSoftware technology and associated services.
In the previous article, we highlighted the business impact of 4D seismic in improving the economics of E&P activities and some of the technical aspects of 4D projects such as feasibility, similarity measures and cross-equalization, and the methods of estimating the optimal time shifts for improving repeatability and 4D interpretation.
In the current article, we will discuss the GeoSoftware 4D capabilities in enabling a quantitative interpretation through different inversion algorithms, allowing for detailed reservoir characterization and discriminating the pressure and saturation effects.
Qualitative 4D Interpretation
The ultimate goal of time-lapse is monitoring the reservoir behavior, locating bypassed areas, improving characterization, mapping pathways, and improving history matching. Qualitative 4D interpretation could be sufficient to meet some of those goals like bypassed/undrained areas, sweep, baffles, flood fronts, contact movement, connectivity, or fault sealing. In those cases we usually tie our interpretation to well logs and dynamic information such as production and injection rates, pressure changes, cumulative production and injection, etc. The tie to production data satisfies many reservoir management objectives and helps resolve some of the uncertainties associated.
Masoud Maleki et al. (2018), studied the challenges of 4D seismic interpretation in the Norne field, Norwegian Sea. The study indicated that acoustic impedance differences derived from a post-stack inversion provided an increase in vertical resolution compared to standard seismic amplitude differences. However, the authors believed a time-lapse pre-stack inversion may improve the interpretation of seismic data and lower the uncertainty associated with separating the fluid and pore pressure effects.
4D Seismic Inversion
The 4D interpretation is usually based on full-stack data (either amplitude or acoustic impedance) as it has better signal-to-noise ratio than partial-stack seismic, and requires less effort, and less time to deliver an interpretation. However, the additional information contained in the pre-stack data could further benefit the 4D interpretation.
Pre-stack 4D inversion would enhance interpretive resolution and provide a better estimate of the changes in elastic parameters from production. Independent measures obtained of time-lapse P-impedance and Vp/Vs ratio (or S-impedance) could be compared with propagation parameters such 4D time shifts and velocity changes (DV/V). In addition, those elastic parameters could be further linked quantitatively to the changes in saturation, pressure or temperature through a calibrated rock physics model.
The most common 4D inversion workflows known to industry include either a separate inversion of the base and monitor survey (independent inversion), an inversion of the difference, or a joint inversion of both base and monitor surveys (Sarkar et al. 2003). GeoSoftware technology has the capability to perform any of those 4D inversion workflows; independent, the difference, or joint inversion.
Figure 1: Map extractions of time-lapse P-impedance and S-impedance over an 8 ms time window around an interpreted Girassol reservoir horizon (after Gonzales-Carballo et al. 2007). The three cross-plots (Top) are respectively taken from data in the vicinity of a water injector, a gas injector, and an oil producer. Calibrating results to the Petro-Elastic Model (PEM) allowed for separating different fluid and pressure scenarios.
It is clear that the conventional seismic signal will only contain part of the information necessary to characterize the reservoir. Some of the information for full bandwidth inversion will have to come from the time-lapse low-frequency response of the subsurface, i.e. the low-frequency difference in the elastic parameter models between monitor and base. Failing to introduce a correct low-frequency model will result in elastic parameter data that includes residual tuning and side lobes. The data will not be correctly calibrated to the well log information and a stacked reservoir might be misinterpreted. Figure 2 shows the effect on the inversion outputs of not using an updated 4D low-frequency model.
For time-lapse studies, two sets of low-frequency models must be considered:
- First, are 3D models that will be used in the inversion of all vintages. Usually, simple interpolation of the well log information will lead to inaccurate elastic parameter models that are biased to a high sand (reservoir) content. Starting with a laterally-constant but structurally-compliant model from a user-editable average over the wells should give an input model for a first-pass inversion that gives a good estimate of the P- and S-Impedance away from well control within the seismic band. Bayesian inference (Jason FFP) can then be used to create a 3D facies model across the project. Next, Facies-Driven Modeling (FDM) is used to create broadband 3D elastic models, from which the low frequencies are used in a second pass of inversion. The low-frequency models, now correctly reflect the 3D elastic parameters and are calibrated to well data, without the need to use a well-log interpolation method.
- The second set of models involves updating the low-frequency models in the time-lapse sense using the estimated time alignment volumes ∆Vp/Vp (assuming the compaction is neglected).
Figure 2: The difference in P-impedance when we don’t update the LFM on the left versus when you update the LFM on the right. The LFM was updated using the time shift from that yellow horizon above the reservoir and that black horizon below the reservoir and distributed this information along the reservoir body. This reservoir production is under pressure maintenance where they inject gas on top and water at the base and produce in between. The blue color (low impedance) is where they inject gas and the yellow color (high impedance) is where inject water. You can see all kinds of artifacts, side lobes and discontinuity due to using the original LFM where on the right-hand side with the updated LFM the side lobes are gone. You can also see that the reservoir is stacked and the blue color is now more continuous.
Peter Mesdag et al. (2015) used a workflow to update the time-lapse low-frequency model in a data-driven manner in time-lapse inversion studies applied to a heavy-oil reservoir produced using the steam-assisted gravity drainage (SAGD) method. The base seismic survey and the difference between the base and monitor surveys were inverted in two separate inversion runs, and no assumptions were made regarding the reservoir changes or the relationships between elastic or reservoir parameters. The effects of production were estimated from the inversion of the difference between the base and monitor surveys and this information was used to update the low-frequency model in the time-lapse sense. He described the workflow as follows:
- Step 1: Full-bandwidth pre-stack inversion of the base survey to provide full-bandwidth inversion models of the reservoir at original conditions.
- Step 2: Inversion of the seismic differences to delineate the steam chambers (volumes where production effects are observed and the low-frequency model needs to be adjusted).
- Step 3: Construction of time-lapse low-frequency models to account for production effects.
- Step 4: Merging of the time-lapse low-frequency models from Step 3 into the time-lapse inversion models from Step 2 to create final full-bandwidth time-lapse models.
Figure 3: Time-lapse inversion workflow to update the low-frequency model. (a) Band-limited time-lapse P-impedance and VP/VS. (b) Same as (a) with the interpreted tops and bases of the steam chambers shown. (c) Low-frequency models for P-impedance and VP/VS. (d) Full-bandwidth P-impedance and VP/VS after merging with the constructed low-frequency models. Note that black in part (c) refers to places where the elastic properties are not affected by production and are assigned a zero value.
Figure 4: Temperature profile compared with inversion. As the elastic parameter changes in the reservoir are dominated by temperature effects, there should be a good correlation between the inversion results and the temperature measurements as illustrated (after Peter Mesdag et al. 2015).
As we know from rock physics, while P-waves are sensitive to changes in both saturation and pressure, S-waves are sensitive to pressure changes. Pressure and saturation effects are more orthogonal to each other in the elastic ∆Ip-∆Is crossplot domain and the ability to discriminate both changes would depend on the quality of attributes driven by the 4D pre-stack inversion. Damasceno et al. (2021 and 2022), Daneshvar et al. (2020) and others demonstrated their effort to discriminate saturation and pressure changes from 4D prestack inversion and how they calibrated the ∆Ip and ∆Is interpretation to a rock physics model.
Figure 5: Comparison between ΔSw, ΔPp, and differential time-shifts (Δt) maps for 4D integrated interpretation over Jubarte field, Offshore Brazil (after Damasceno et al. 2022).
Rock-physics 4D inversion
As 4D technology evolves and data quality improves, the possibility to invert directly for changes in pressure, saturation, compaction, and fluid compressibility from 4D seismic, could facilitate the tie of seismic data to production data and help us to refine reservoir-model history matching (David Johnston 2013).
Rock-physics-based 4D inversion relies on a calibrated model that can predict the changes in the elastic properties that result from saturation and pressure changes. GeoSoftware’s patented technology (Jason RockMod 4D) performs simultaneous geostatistical inversion of two or more seismic vintages to invert directly for changes in pressure and saturation. It is a Bayesian and Markov Chain Monte Carlo (MCMC) engine and it has the following features:
- A joint inversion of facies and properties
- Uses all seismic vintages simultaneously
- Invert directly for the change in pressure and saturation
- Honor the rock physics models and incorporate the associated uncertainty
- Facies modeling and nesting are included
- Performs in either the time or depth domains
- Connects to reservoir grids
Figure 6: A schematic of Jason Rock-physics 4D inversion (RockMod 4D).
Jason RockMod 4D inverts all vintages simultaneously. The simultaneous geostatistical inversion perturbs the properties and facies to estimate the reservoir properties. Porosity, clay content, and saturation are typically the primary properties being inverted. In the reservoir, permeability is computed from porosity using empirical relationships per facies. The base saturation is computed from the theoretical capillary functions (one per lithofacies). The base pressure is calculated from 1D pore pressure analysis. The expected changes in pressure and saturation are modeled from well logs, and production data through rock physics modeling (RPM). The modeled probabilities for these expected changes are prior to the inversion. The priors for the base properties are defined by fitting data from the well logs. The monitor properties are the sum of the base properties and delta properties. Building/obtaining a reliable rock physics model (RPM) is a critical part of the Rock-physics inversion workflow. The RPMs are used during the inversion to go from properties to seismic via forward modeling. Synthetic of base and monitor honor the associated seismic of base and monitor.
The algorithm always accepts realizations that increase the posterior pdf value, and only sometimes accepts downward steps. The process continues updating until the probability is no longer changing. All realizations are equally possible as they honor all input data (seismic and prior information).
Jason RockMod 4D unlocks the quantitative information unavailable to traditional 4D inversions. It provides probabilistic outputs and facilitates capturing uncertainty by delivering scenarios of facies and reservoir properties at a resolution necessary for geomodelling. It makes it easy to update models once new information is available, therefore tracking production-related changes or inefficient production or injection processes. RockMod 4D could also handle different data types including PP-PS and azimuthal seismic.
Summary
Here are some takeaways from today’s article:
- The qualitative 4D interpretation could be sufficient to meet some of reservoir management goals like bypassed/undrained areas, sweep, baffles, flood fronts, contact movement, connectivity, or fault sealing. However, a more quantitative interpretation could be required for some of the challenging 4D projects.
- The additional information contained in the pre-stack 4D inversion would enhance the interpretive resolution and provide a better estimate of the changes in elastic parameters caused by production. Independent measures of time-lapse P-impedance and Vp/Vs ratio (or S-impedance) could be compared with propagation parameters such 4D time shifts and velocity changes (DV/V). The 4D inversion when calibrated to the rock physics model would be effective in distinguishing saturation changes from pressure changes.
- Failing to introduce a correct 4D low-frequency model in 4D inversion, (the low-frequency difference in the elastic parameter models between monitor and base) will result in elastic parameter data that includes residual tuning and side lobes. This would impact the quality of the 4D interpretation and the stacked reservoirs might be misinterpreted.
- Jason rock-physics 4D inversion (RockMod 4D) is a joint inversion, that honors the rock physics model and inverts all vintages simultaneously to directly estimate the changes in pressure and saturation. RockMod 4D unlocks the quantitative information unavailable to traditional 4D inversions and facilitates capturing the uncertainty by delivering scenarios of facies and reservoir properties at a resolution necessary for geomodelling.
To learn more about how GeoSoftware's reservoir experts can help you with your next 4D project, visit our Services page.
AUTHOR: Mohammed Ibrahim, Global Services Manager
Mohammed has over 20 years of experience in the oil and gas industry, most notably in geostatistical seismic inversion, QI geophysics, 4D seismic, and azimuthal seismic analysis. He has a proven record in integrating large, multi-scale, multi-disciplinary data to characterize hydrocarbon reservoirs and build “what-if” scenarios for both exploration and production fields. Mohammed has worked globally with NOCs, IOCs, and super-major oil and gas companies in a wide variety of complex petroleum provinces in North and Latin America, the Atlantic margins, the Middle East, and Western Australia. Mohammed holds a Ph.D. degree in geophysics from Michigan Technological University, and he currently oversees the professional services function within GeoSoftware.